May 16, 2025 | Blog Post
Significant advancements have been made in the therapeutic landscape of cancer, driven by the imperative to develop treatments for patients that are both effective and less toxic. Traditional therapeutic strategies like surgery, chemotherapy, and radiation therapy are generally effective but inherently limited by their lack of specificity regarding the molecular profile of tumors and collateral damage to healthy tissues. These constraints have spurred the pursuit of innovative approaches that leverage the body’s intrinsic defense mechanisms, collectively called immunotherapies. Immunotherapy activates the immune system to recognize and eradicate cancer cells and signifies a paradigm shift in oncology. By enhancing the body’s anti-tumor immune defense, immunotherapy has broadened the therapeutic arsenal and redefined the trajectory of cancer care, offering the potential for durable responses and improved patient outcomes.
The conceptual foundation of immunotherapy dates back over a century, with pioneering observations made by Dr. William Coley in the late 1800s. Coley noted tumor regression in patients with concurrent bacterial infections, suggesting that immune system activation could combat malignancies. Despite the initial promise, his methods, which involved administering bacterial toxins, lacked the mechanistic insights that modern science provides. Advances in immunology throughout the 20th century elucidated key components of the immune response, including T-cells and cytokines, and laid the groundwork for contemporary immunotherapeutic strategies. Checkpoint inhibitors emerged in the early 21st century; these block inhibitory pathways on T-cells that are a safety mechanism to prevent autoimmunity. Ipilimumab (which targets CTLA-4) and nivolumab/pembrolizumab (which target PD-1) showed that blocking immune checkpoints can reinvigorate anti-tumor immunity.
Immunotherapy encompasses several distinct modalities operating through different mechanisms. As mentioned above, checkpoint inhibitors disrupt inhibitory signals that cancer cells exploit to evade immune detection, thereby reinvigorating T-cell activity. Chimeric antigen receptor (CAR) T-cell therapy involves genetically engineering T-cells to express synthetic receptors that recognize tumor-specific antigens, enabling tumor clearance. Monoclonal antibodies bind to surface proteins on cancer cells, tagging them for destruction or blocking proliferative signaling. Cancer vaccines prime the immune system to recognize tumor-specific antigens, while cytokine therapies such as interleukin-2 and interferons amplify immune responses, enhancing tumor eradication.
Non-small cell lung cancer (NSCLC), the most common subtype of lung cancer, has experienced significant advancements due to immunotherapy. Drugs like pembrolizumab and nivolumab have become the standard of care, particularly for tumors with high PD-L1 expression. These therapies promote T-cell activation by disrupting the PD-1/PD-L1 axis, fostering more robust and sustained anti-tumor immune responses. Clinical trials have demonstrated that immunotherapy often surpasses chemotherapy in both efficacy and tolerability, representing a paradigm shift in the management of NSCLC.
Although immunotherapy has achieved notable successes, its effectiveness is inconsistent across all cases, making real-time monitoring of therapeutic responses essential for optimizing clinical outcomes. Traditional imaging techniques may fall short in promptly and accurately portraying therapeutic responses, especially in patients with heterogeneous responses or stable disease. Liquid biopsies have emerged as a minimally invasive alternative, analyzing circulating tumor DNA (ctDNA) molecules shed by tumor cells into the bloodstream. Longitudinal tracking of fluctuations in ctDNA levels enables clinicians to assess systemic tumor burden and treatment efficacy precisely. These approaches can elucidate the heterogeneity of immunotherapy responses and facilitate timely therapy modifications. Concurrently, monitoring T-cell activation and differentiation patterns provides insights into the immune system’s engagement, revealing proliferation, exhaustion, and memory formation markers correlating with differential therapeutic outcomes or the emergence of immune-related toxicities.
In conclusion, immunotherapy has redefined the field of oncology, harnessing the immune system to achieve targeted and durable anti-tumor responses. Its success is exemplified by breakthroughs in NSCLC treatment, where checkpoint inhibitors have improved clinical outcomes compared to traditional chemotherapy for many patients. However, timely and accurate capture of therapeutic response remains a challenge. Liquid biopsies and immune cell population profiling represent pivotal advancements in combating this challenge. Ongoing research is honing these technologies, enabling synergies between immunotherapy and real-time molecular monitoring of treatment response, ultimately leading to improved outcomes with precision immuno-oncology.
References:
- Pardoll, D.M. (2012). The blockade of immune checkpoints in cancer immunotherapy. Nature Reviews Cancer, 12(4), 252-264.
- Topalian, S.L., et al. (2012). Safety, activity, and immune correlates of anti-PD-1 antibody in cancer. New England Journal of Medicine, 366(26), 2443-2454.
- Ribas, A., & Wolchok, J.D. (2018). Cancer immunotherapy using checkpoint blockade. Science, 359(6382), 1350-1355.
- June, C.H., O’Connor, R.S., Kawalekar, O.U., Ghassemi, S., & Milone, M.C. (2018). CAR T cell immunotherapy for human cancer. Science, 359(6382), 1361-1365.
- Aredo J.V. et al. Liquid Biopsy Approaches for Cancer Characterization, Residual Disease Detection, and Therapy Monitoring. Am Soc Clin Oncol Educ Book 45, e481114 (2025). DOI:10.1200/EDBK-25-481114
- Anagnostou, V., Ho, C., Nicholas, G. et al. ctDNA response after pembrolizumab in non-small cell lung cancer: phase 2 adaptive trial results. Nat Med 29, 2559–2569 (2023). https://doi.org/10.1038/s41591-023-02598-9
May 16, 2025 | Blog Post
Immunotherapy, in the form of immune checkpoint inhibition (ICI), has transformed cancer care across multiple tumor types. However, existing biomarkers often fail to accurately predict which patients will draw maximal benefit. Tumor Mutation Burden (TMB) has emerged as a tumor-agnostic predictive biomarker of immunotherapy response. Measured as the number of somatic mutations per megabase (mut/Mb) of analyzed DNA sequence, TMB serves as a proxy of tumor “foreignness” to the immune system. The U.S. Food and Drug Administration (FDA) has granted approval of ICI with pembrolizumab for tumors with TMB ≥ 10mut/Mb regardless of cancer type. Despite this milestone, its clinical utility as a pan-cancer companion diagnostic for ICI is limited and TMB remains imperfect as it does not effectively and consistently predict immunotherapy response. In our recent publication (DOI), we revisit the biological, technical, and clinical nuances that limit TMB’s value and highlight how its fine-tuning might overcome these challenges.
The biological premise of TMB is straightforward: more mutations = higher chances of tumor immune recognition. But this view oversimplifies a process with a high degree of complexity. Not all mutations translate into peptides, and not all peptides are immunogenic. Only a fraction of mutation-associated peptides, known as neoantigens, can be effectively presented on major histocompatibility complex (MHC) molecules on cell surface and be recognized by T-cell receptors. Hence, neoantigen quality matters more than mutation quantity. A tumor with high density of immunogenic mutation-associated neoantigens may therefore respond better to ICI than a tumor with higher TMB but poorer neoantigen quality.
TMB is a static snapshot, while cancer is a moving target. Over the course of tumor evolution, immunotherapy imposes selective pressure and bottlenecks, causing cancer clones harboring different TMB values to be eliminated or prevail through a process known as immunoediting. This Darwinian process can significantly alter tumor’s neoantigen landscape over time. Thus, TMB as a numeric or binarized value (high/low) falls short in capturing the dynamic nature of tumor evolution.
In addition, not all mutations carry the same significance when it comes to predicting immunotherapy responses. Some happen as early as the initial steps of carcinogenesis, are shared among many cancer clones and are termed as “clonal”. Others, known as “subclonal”, occur later, are shared only among few tumor cells and are more prone to loss over time. Taking a step beyond tumor architecture through a more functional scope, our group has previously shown that the genomic context of mutations plays its own role in shaping their likelihood of loss due to immunoediting. Mutations occurring in the multicopy state or found in haploid genomic regions are less likely to be eliminated. Mutations in multiple copies may be biologically protected from loss, while mutations in essential haploid genomic regions cannot be purged without compromising cell survival (DOI).
Another important consideration lies in the specimen and methodology employed to calculate TMB. Current FDA approval refers to tissue-based TMB calculation through next-generation sequencing (NGS) of a prespecified gene panel. However, this method is subject to biases related to tumor heterogeneity, sampling site, and tumor purity, especially in metastatic or heavily pretreated settings. In our piece, we touch upon the opportunities blood-based TMB estimation provides. Our paper discusses the emerging role of blood-based TMB estimation from circulating tumor-derived DNA fragments (ctDNA). Liquid biopsy offers a less invasive, real-time alternative that captures spatial and temporal heterogeneity, enabling real-time tracking of tumor mutational status throughout ICI treatment.
The approved cutoff of 10mut/Mb for ICI administration, although convenient, may not be universally applicable in the pan-cancer context. Tumor histology and lineage play a critical role in shaping mutation patterns and immune responses. For instance, malignant mesothelioma typically stands in the lower TMB spectrum, yet some patients respond to ICI. Alternative biomarkers, such as genome-wide loss of heterozygosity, may prove more useful in these contexts (DOI). Our published work includes an analysis of TMB values in correlation with ICI responses across several cancers, highlighting the discrepancies histology and lineage impose when it comes to establishing cutoffs that maximize the predictive value of TMB.
Furthermore, the overall tumor genomic landscape within which TMB is interpreted holds great significance. High TMB may result from mutagenic processes such as microsatellite instability or POLE/POLD1 mutations (ultra-mutated phenotype), which are associated with favorable ICI responses. Additionally, distinct mutational signatures, including those induced by ultraviolet light, tobacco smoke, or APOBEC enzymes, can influence immunogenicity. Finally, co-mutation patterns largely dependent on cancer lineage add an extra biological modifier of ICI response that needs to be considered when calibrating TMB through the lens of tumor biology. Example of such patterns include STK11/LKB1 mutations, which have been shown to dampen ICI response despite high TMB.
Overall, it is true that TMB is far from perfect when it comes to predicting ICI response. Looking into the future, its biological and technical standardization is expected to upgrade its predictive worth and allow for maximal leverage of its value in the clinical setting.
Jan 7, 2025 | Blog Post
Precision oncology is revolutionizing the way we treat cancer by tailoring therapies to the genetic makeup of individual tumors. As our understanding of cancer’s molecular biology continues to expand, therapies are becoming more targeted, effective, and individualized. This shift toward personalized medicine is gradually becoming the standard of care, offering hope for better outcomes in patients with advanced cancers.
Molecular Tumor Boards (MTBs), are multidisciplinary teams of experts that play a pivotal role in the application of precision oncology and the translation of research findings into clinical practice. The Molecular Tumor Board routinely reviews cases of individuals with advanced cancer and interprets the genomic data obtained through next-generation sequencing (NGS) to ultimately explore molecularly-informed treatment recommendations. These MTBs however, are most commonly available in tertiary cancer centers, making access to them still limited. That is why our team at the Johns Hopkins Molecular Tumor Board (JH MTB) recently published a case report (DOI) in The Journal of Clinical Oncology (JCO), to open up an educational discussion on the genomically informed treatment paradigm. This case report leverages a discussion held at our Molecular Tumor Board that highlights the important role of the MTB in the management of metastatic breast cancer, and it details the journey of a patient whose tumor developed resistance to targeted therapy, identified using NGS, and interpreted by the JH MTB.
We discussed the case of a young woman diagnosed with early stage hormone receptor–positive, HER2-negative breast cancer, who as per standard of care, received neoadjuvant chemotherapy followed by bilateral mastectomy. Germline testing identified a heterozygous pathogenic loss-of-function PALB2 frameshift mutation which is known to be associated with an increased lifetime risk of breast, pancreatic, and ovarian cancer. The patient’s cancer recurred four years after the initial diagnosis and as biopsy of a metastatic site showed hormone receptor–positive breast cancer, she received letrozole monotherapy. Following further disease progression 15 months later she received the PARP inhibitor olaparib as part of a clinical trial given the presence of the PALB2 germline mutation.
PALB2 is one of the genes involved in DNA repair, specifically in homologous recombination (HR). Loss of function of PALB2 leads to the inability to properly repair DNA, specifically double-strand breaks, which can result in genetic instability and contribute to cancer progression. This is referred to as homologous recombination deficiency (HRD), and, as in this case, it can be driven by loss-of-function mutations in one of the genes of the HR pathway. Cancers with DNA repair deficiency rely on alternate DNA repair mechanisms and this creates an opportunity for therapies that block these alternate DNA repair pathways such as PARP inhibitors (PARPi) to block DNA repair in cancer cells ultimately leading to tumor cell death.
However, some tumors can develop resistance to PARPi through acquired mutations that restore homologous recombination, like in this case. At the time of disease progression on olaparib, a liquid biopsy detected the germline PALB2 mutation, together with a plethora of new PALB2 mutations that all restored the PALB2 reading frame, practically negating the effect of the germline loss-of-function mutation. The JH MTB reasoned that these reversion mutations all restored homologous recombination that allowed the metastatic clones to escape synthetic lethality through PARPi therapy. A large number of different PALB2 reversion mutations were detected in the bloodstream, which represents the reservoir where circulating DNA from any metastatic site is shed. As such, the JH MTB team hypothesized that different PALB2 reversion mutations were acquired and selected for in various metastatic sites and potentially at different timepoints during PARPi therapy. This essentially means that these reversion mutations, although all driven by a shared selective pressure of PARPi therapy, emerged separately at different metastatic sites and at different times during tumor evolution.
The emergence of polyclonal reversion mutations in PALB2, following PARP inhibition, suggest convergence evolution in this tumor, through independent genomic events, in response to the shared selective pressure of target therapy, ultimately restoring homologous recombination and DNA repair, and leading to a clinically refractory phenotype. Such reversion mutations have been observed in ovarian, breast, pancreatic, and prostate cancer with a prevalence of approximately 26% and the cancer clones harbouring these reversion mutations gain a fitness advantage, followed by positive selection that clinically manifests as therapy resistance. As seen in this case, different subclones can develop genomically distinct but phenotypically similar resistance when subjected to a shared selective pressure.
This case highlights the importance of elucidating the underlying mechanism of resistance and uncovering the molecular biology driving the refractory phenotype. By leveraging advanced genomic techniques like next-generation sequencing and the multi-disciplinary expertise of Molecular Tumor Boards, we can uncover the intricate mechanisms behind treatment resistance, such as the polyclonal reversion mutations observed in this patient. Our findings also illustrate the importance of real-time monitoring to adapt treatment approaches. As personalized therapies continue to evolve, the integration of genomic profiling into clinical decision-making remains essential for identifying the most effective treatment options and overcoming resistance. This case additionally highlights the advantage of liquid biopsy to both allow for minimally invasive real-time serial monitoring for arising resistance variants and to enable comprehensive assessment of the genomic landscape across metastatic sites in heterogeneous tumors. The collaborative, multidisciplinary MTB review process exemplified by this case is a powerful model for translating molecular insights into actionable treatment strategies, ultimately improving outcomes for patients with advanced cancer. Lastly, we emphasise the need for broadening access to MTBs, ensuring that genomically informed care is available to a wider range of patients.
Nov 20, 2024 | Blog Post
Posing hypothesis-driven and clinically relevant questions represents one of the pillars of scientific discovery that can be translated in interventions that may improve patient outcomes. And that’s exactly what our research team was able to do with BR.36; a multi-center, randomized, ctDNA-directed, phase 2 trial of molecular response-adaptive immuno-chemotherapy for individuals with lung cancer. Recently published in Nature Medicine (DOI), and building on years of work on minimally invasive liquid biopsies in capturing therapy response to immunotherapy, our group formed, asked, and investigated the clinical value of using a molecular readout of therapy response during immunotherapy.
Evaluation of therapy response on immunotherapy represents a challenge. Imaging that is predominantly used to evaluate cancer’s response to treatment, often falls short in rapidly and accurately capturing the therapeutic effect. The limitations of imaging are intensified in patients with radiographic stable disease or mixed responses, both representing heterogenous and hard to assess patient groups. In addition, already existing snapshot tumor-based biomarkers such as PD-L1 expression and tumor mutation burden (TMB), that can help guide therapy selection, are imperfect and inconsistently predict clinical outcomes with immunotherapy.
That’s where liquid biopsies and analyses of cell-free circulating tumor DNA (ctDNA) have gained momentum and can be particularly informative. As cells in our body die, they shed fragments of their DNA in the bloodstream. Cancer cells do so as well and these cell-free circulating tumor DNA remnants can be picked up by liquid biopsies (LB). From a LB assay standpoint, cell-free DNA is isolated from blood, followed by ultra-sensitive techniques that allow us to focus on specific regions of DNA and look for changes in the genetic code, known as mutations. These minimally invasive, innovative approaches allow for real-time tracking of circulating tumor burden, thereby molecularly assessing cancer’s response to treatment.
After identifying mutations in ctDNA, we are faced with the challenge of deciphering which of the mutations detected by the LB are truly tumor-derived. Here comes another challenge, that of identifying which mutations are coming from cancer cells and which ones come from other tissues and as such represent “biological noise”. These confounding mutations can be germline, meaning mutations passed down from previous generations, or can be originating from blood cells, which is termed “clonal hematopoiesis” (CH). To solely focus on tumor-derived mutations in our study, we used matched normal DNA genomic data, obtained through next generation sequencing of white blood cells (WBCs), which in turn allowed for filtering out CH-derived and germline mutations.
With that background in mind, let us dive deeper into the fundamental questions posed. Starting with the definition: What is ctDNA response? How soon after immunotherapy initiation should we look for it? How concordant is ctDNA molecular response with imaging response? And how does it relate to survival and clinical outcomes? Are there specific patients that would benefit most from a molecular assessment of their cancer’s response?
Let us rewind and unfold these one by one. Fifty patients with metastatic non-small cell lung cancer (NSCLC) were enrolled in the first observational phase of our trial and received immunotherapy as standard of care. LBs were performed with each of the first three cycles of immunotherapy and ctDNA load was estimated. Molecular response (mR) was defined as clearance of ctDNA mutation levels, whereas persistence or rise of ctDNA levels was classified as molecular disease progression (mPD). Our findings reveal that the median time necessary for achievement of mR was 2.1 months, or in other words, after 2 cycles of immunotherapy. We found that most patients with radiographic complete (CR) or partial (PR) response prior to cycle 3 of immunotherapy had mR on their liquid biopsy testing. We additionally and importantly found a strong correlation between molecular response and improved clinical outcomes, as patients in the mR group attained longer overall survival (OS) and progression-free survival (PFS) than patients showing mPD.
These findings suggest a unique actionable opportunity to use molecular response assessment to more optimally decipher the true therapy response including the heterogeneous group of patients with radiographically stable disease. Molecular responses were largely concordant with radiographic responses, however the former were more informative and effective in predicting clinical outcomes and survival.
Upon investigating these questions, what is next? Clinical translation. Eager to implement the aforementioned findings in clinical practice, we designed the second phase of BR.36; a ctDNA molecular response-driven interventional randomized stage of the trial that will assess the value of ctDNA molecular response in guiding therapeutic decision making (NCT04093167) and inform which patients should receive immunotherapy monotherapy vs. a combination of immunotherapy with chemotherapy. Looking at the expanding landscape of immunotherapy treatments for individuals with metastatic lung cancer, our vision is that ctDNA molecular response can rapidly and accurately predict therapy response and allow us to navigate treatments, tailoring therapies to the right patient populations at the right time.
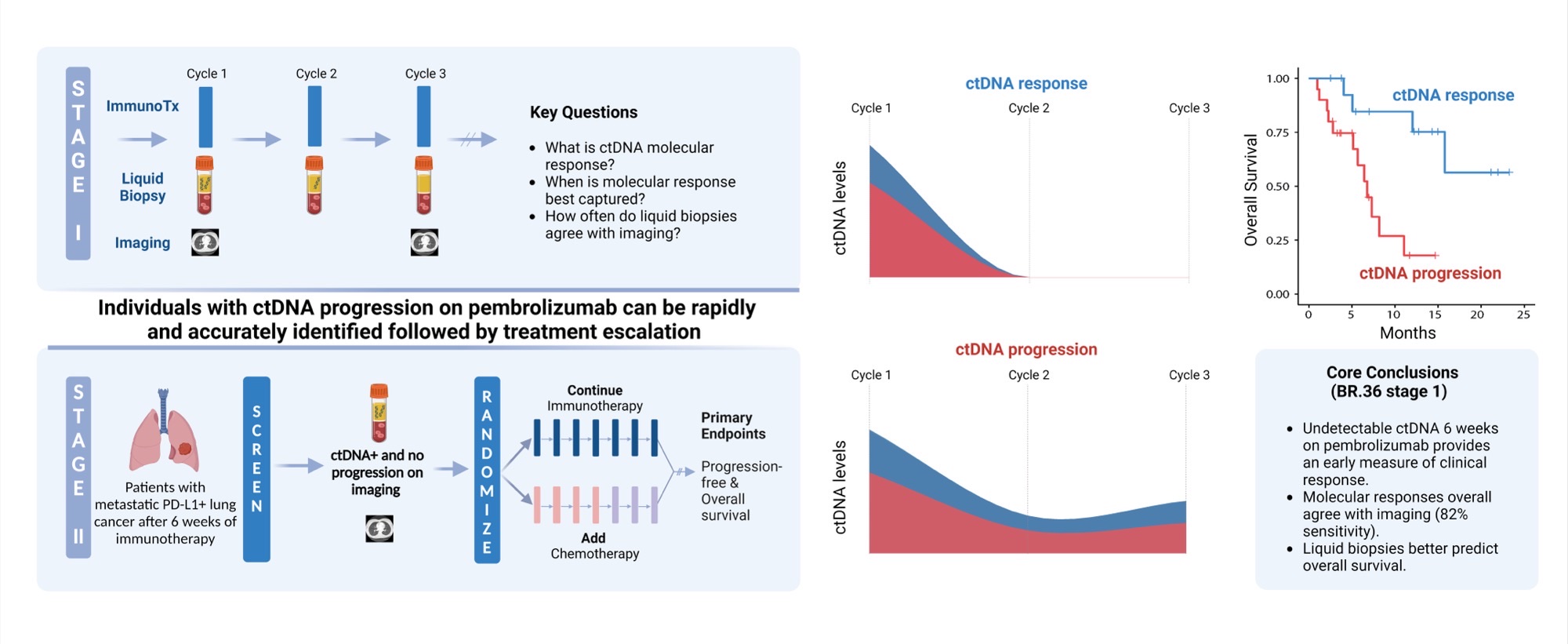
Interested in more reading? Here’s a list of liquid biopsy studies from our group:
Neoadjuvant nivolumab or nivolumab plus LAG-3 inhibitor relatlimab in resectable esophageal/gastroesophageal junction cancer: a phase Ib trial and ctDNA analyses. (DOI)
Liquid biopsy approaches to capture tumor evolution and clinical outcomes during cancer immunotherapy. (DOI)
Elucidating the Heterogeneity of Immunotherapy Response and Immune-Related Toxicities by Longitudinal ctDNA and Immune Cell Compartment Tracking in Lung Cancer. (DOI)
Apr 4, 2024 | Blog Post
How do we predict which patients with lung cancer will attain favorable clinical outcomes with immunotherapy? Our research focuses on answering this challenging question through assessing the dynamics of circulating tumor DNA (ctDNA) during immunotherapy in patients with metastatic non-small cell lung cancer (NSCLC).
Oncologists use predictive and prognostic biomarkers – clues that guide choice of therapy and predict clinical outcomes, such as PDL1 expression and tumor mutation burden (TMB). These tumor tissue-based biomarkers, however, do not always predict clinical outcomes. In our recent study published in Clinical Cancer Research (https://doi.org/10.1158/1078-0432.CCR-23-1469) our group investigated blood-based characteristics of clinical response and immune-related adverse effects (irAEs) for individuals with lung cancer.
But let’s backtrack for a second. It’s important to note that a sizable fraction of patients with metastatic NSCLC receive an immunotherapy-containing regimen, introducing an unmet need to rapidly and accurately identify which patients are most likely to do well on immunotherapy – and potentially spare them of unnecessary interventions – as well as which patients are at higher risk for disease progression, necessitating further interventions
Physicians conventionally and predominantly use imaging techniques to assess a tumor’s response to treatment. These methods, however, have technical limitations regarding the level of detection, as imaging cannot capture microscopic disease. In addition, imaging may be less effective in capturing heterogenous responses with immunotherapy which can impact patients whose cancer appears to be stable on imaging. The challenge here is identifying the true disease status of these patients.
Liquid biopsies (LB) and analyses of circulating cell-free tumor DNA (ctDNA) have emerged as groundbreaking technologies aiming at addressing and tackling these challenges. These methods offer a minimally invasive alternative to traditional tissue biopsies. But what exactly is ctDNA? As most tumors rapidly divide, necrosis and apoptosis occur. This essentially means that cancer cells die. When that happens most cancer cells “shed” small fragments of their DNA in the bloodstream. Scientists are able to isolate and detect these “cell-free” DNA fragments through LBs. Here’s how it works in simple terms: they take a small sample of blood, separate the liquid part (plasma), and then pick out the tumor’s circulating DNA from it. After that, they sequence it, meaning they zoom in on the ctDNA and read through it, looking for any genetic changes in the building blocks of the DNA, known as mutations.
Through liquid biopsies, we can estimate the cell-free tumor load (cfTL), meaning how much ctDNA was detected in the bloodstream, as well identify specific mutations in ctDNA. Through this process, physicians are able to see whether molecular progression is occurring behind the scenes in patients with radiographic stable disease. This would appear as persistence or rise of ctDNA levels on LB during immunotherapy. Another way this could manifest is as emergence of specific mutations detected on LB that can be characterized as mechanisms of primary or acquired resistance to treatment.
Based on clearance or persistence of cfTL, patients in our study were mainly placed into one of two groups – the molecular response group (mR), or the molecular progressive disease group (mPD). Importantly, our study found that molecular responses were associated with longer survival, highlighting the potential of ctDNA dynamics as a predictive tool for treatment outcomes. Patients in the mR group attained longer overall survival (OS) and progression free survival (PFS) than patients in the mPD group. Interestingly, a correlation was observed between lower ctDNA levels at baseline and more favorable clinical outcomes for patients receiving single-agent immunotherapy.
But what about a negative LB result? What does it mean when ctDNA is not detected? Can we confidently trust this result and de-escalate treatment? Despite the advances made in the technology behind LBs, there are still significant limitations that make physicians air on the side of caution when making therapeutic decisions based on negative LB results.
On the one hand, are the technical limitations of LBs, which mainly revolve around the level of detection, and the specifics behind how ctDNA is isolated and sequenced, which we call “technical” noise. On the other hand, LB results can often be confounded by “biological” noise. This refers to some mutations identified in liquid biopsies that are not truly tumor-derived and could lead to a false positive result. Some of the mutations picked up on LBs do not come from the tumor, but from blood cell precursors. This phenomenon is called clonal hematopoiesis (CH). Understanding which mutations are from the tumor itself is a big challenge that we addressed in our study through additionally sequencing matched normal DNA from white blood cells. This allowed our research team to exclude CH mutations and identify tumor-derived mutations.
Aside from assessing patient outcomes to immunotherapy, we additionally investigated the role of LBs in detecting clues that may predict toxicity. To do so, we evaluated immune cell repertoire dynamics in relation to clinical response and the emergence of immune-related toxicities. Through T-cell receptor (TCR) sequencing, we were able to see which TCR clones were increasing, and which were decreasing between baseline and on-treatment timepoints. These TCR clones were then clustered based on similarity. Significant expansions and regressions of specific TCR clusters were observed in patients who developed immunotherapy toxicity. These patients were also found to have elevated plasma protein expression of pro-inflammatory mediators, both at baseline and during treatment. Monitoring T cell dynamics and plasma proteomic profiles could help identify patients at higher risk of severe toxicities early on, allowing for timely intervention.
Taken together, our research group concludes that despite its limitations, ctDNA-based molecular response is a robust predictor of clinical outcomes in patients with lung cancer receiving immunotherapy and can be particularly informative for patients with stable disease on imaging. We anticipate that using liquid biopsies to longitudinally track ctDNA as well as T cell repertoire monitoring, could enhance clinical decision-making and improve patient outcomes during immunotherapy. Our work has already been implemented in ctDNA-driven clinical trial design for patients with lung cancer receiving immunotherapy (https://doi.org/10.1038/s41591-023-02598-9, NCT04093167).